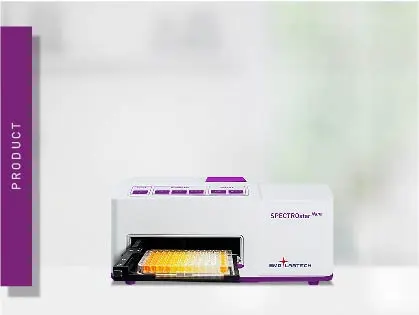
SPECTROstar Nano
Absorbance plate reader with cuvette port
Scientists have cultured micro-organisms for many years in liquid media, measuring cell proliferation in a cuvette using absorbance at 600nm.
The use of microplates in formats up to 96 well has provided the means to measure multiple conditions simply and easily in the appropriate microplate reader. The advent of microplate readers with temperature control and shaking options has provided researchers with the possibility of long-term studies requiring no user interaction, other than to load the plate and analyze the data. Microplate readers that offer the capacity to read in multiple modes, absorbance, fluorescence, and luminescence, provide further flexibility in the potential experiments that can be performed by enabling the assessment of gene expression or cell activity in relation to growth, for example.
Microbes that have no specific atmospheric requirements for growth will be happy in the standard environment of the microplate reader. Those requiring more specific oxygen or carbon dioxide conditions may grow but not in a representative or healthy manner, potentially compromising the experiment. The Atmospheric Control Unit (ACU) from BMG LABTECH provides the researcher with a system that uniquely enables control of both the oxygen and carbon dioxide concentrations in an independent manner.
Microbes that have been demonstrated to grow well in the BMG LABTECH readers without the need for gas control are Salmonella enteric (AN140), Vibrio fischeri (AN199), Candida albicans (AN189), and Corynebacterium glutamicum (AN125).
However, more fastidious microbes require elevated CO2 levels as clearly shown with Neisseria meningitides (AN155) that thrive in a 5% CO2 environment. In addition, Campylobacter jejuni has even more stringent requirements for healthy growth, 42°C with 7% O2 and 10% CO2 (AN 217), with similar stringent growth requirements for Helicobacter.
The ability to effectively grow microbes in optimum conditions allows for the possibility to design experiments to investigate many parameters, including antibiotic resistance minimum inhibitory concentrations. The design of the ACU allows the researcher to simply define the O2 and CO2 concentrations required, without the need to change gases or to use expensive premixed gases on different days for different microbes.
Absorbance plate reader with cuvette port
Powerful and most sensitive HTS plate reader
Most flexible Plate Reader for Assay Development
Upgradeable single and multi-mode microplate reader series
Flexible microplate reader with simplified workflows
Antimicrobial resistance is a formidable problem across the world. Find out how microplate readers can help tackle resistant microbes.
You want to make sure that your cell culture is free of mycoplasma? BMG LABTECH microplate readers are the perfect measurement platform to read MycoAlert® mycoplasma detection kits.
OD600 is part of a lot of microbiology studies. Reason enough for us to use this article to explain why the method is used so intensively and what should be considered for the measurement.
Obligate anaerobic gut bacteria require an absolute anaerobic environment. Read how the FLUOstar Omega was used to develop new methods to study the human gut microbiome.