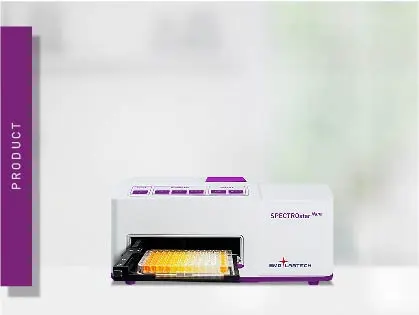
SPECTROstar Nano
Absorbance plate reader with cuvette port
For many years, binding assays have been used extensively for fundamental biological research and are a key part of pharmacology and medicinal chemistry. Affinity binding assays are typically used to determine the strength of binding of a ligand (protein, peptide, or small molecule drug) to a target biomolecule. Therefore, many experiments are aimed at determining the binding affinity of potential binding partners represented by the equilibrium dissociation constant KD, the concentration of ligand at which half the binding sites on a biomolecule are occupied at equilibrium. The target biomolecule could be another protein, antibody, DNA, RNA, G-protein coupled receptors, kinases, or a multitude of other receptors, which when activated by a ligand, produce a pharmacological effect in the body.
Once an affinity binding assay has been established for a ligand/biomolecule pair, competitive binding assays can be developed. Competitive binding assays allow the high-throughput screening of combinatorial or natural compound libraries. This can be used to develop drugs that can interfere with downstream events effected by activation of the target biomolecule by endogenous ligands. Binding assays are also routinely used in finding and defining interaction partners and interactions linked with diseases. In this article, I will attempt to give a brief overview of commonly used or interesting binding assays using the different detection modes available on microplate readers.
Binding assays rely on the quantitative/qualitative detection of binding partners as well as the resulting binding complexes. For this purpose, either the target biomolecule, ligands or both need to be labelled. A plethora of labelling/detection techniques are used to perform binding assays including radioligand assays, affinity chromatography, surface plasmon resonance, isothermal titration calorimetry and light-based techniques using absorbance, fluorescence, or luminescence readouts.1 This review will focus on binding assays based on light detection/labelling techniques commonly used in microplate readers.
A great number of binding assays, using microplate readers for detection of a light-based readout, have been described in the literature or are available in kit form. Light detection modes in common use are: absorbance, fluorescence, luminescence, FRET (Fluorescence Resonance Energy Transfer), Fluorescence polarization (FP), TRF (time-resolved fluorescence), TR-FRET (time-resolved fluorescence energy transfer), and AlphaScreen/AlphaLisa. For a detailed description of these strategies, including a description of how binding affinity parameters can be determined, see also: How to determine binding affinity with a microplate reader.
In general, the techniques mentioned above can all be used in affinity binding assays to determine KD, or in competitive binding assays for drug screening. However, they markedly differ in sensitivity, ease of setup and cost. In this article, detection techniques are listed in increasing order of relative sensitivity and examples will be provided to highlight the advantages and disadvantages of each. Sensitivity, in a binding assay is commonly determined by calculating the limit of detection (LOD), defined as the minimum concentration of labelled ligand/complex that can be detected over the blank. Although the LOD value is very specific to each individual assay, general relative trends can be ascribed to each technique mentioned below.
Absorbance-based binding assays
Absorbance is simply the measurement of the intensity of light, at a particular wavelength, that passes through a sample as compared to air. The absorbance measurement (A) is therefore a ratio and in particular A = -Log Isample/Iair. As many compounds absorb light, impurities in the assay mixture can interfere with the compounds you are trying to detect in a binding assay, giving rise to a high background signal. Thus, it is generally advised to use purified reagents or include multiple washing steps in your absorbance-based binding assays. Despite these drawbacks, absorbance-based binding assays are low cost as no labelling is required for detection of a chromophore.
The most common absorbance-based binding assays are ELISA (Enzyme Linked Immunosorbent Assay) assays. Absorbance ELISA binding assays utilise antibody pairs specific for the tested ligand and target biomolecule. While one antibody is used to bind the biomolecule or ligand to the microplate surface, the other binds the other binding partner. The binding complex is detected by a colorimetric enzymatic reaction coupled to the second antibody, while any unbound binding partners in solution are removed by multiple washing steps. Traditional ELISA assays take a great deal of time (3-5 hrs) due to washing steps, in part to ensure that the absorbance readout does not have high background noise. However, more and more absorbance-based ELISA kits are being developed that streamline the handling process by requiring only a single wash step during sample preparation.
Fluorescence-based binding assays
Very few compounds in nature fluoresce at visible wavelengths. Labelling the ligand or biomolecule in a binding assay with a fluorescent tag can significantly increase the sensitivity of an assay over an absorbance-based one and in general, a better limit of detection and signal linearity over a much larger concentration range can be achieved. Furthermore, fluorescent labelling of small peptides and proteins can be easily achieved in most cases by using simple, inexpensive reagents.
A serious drawback to the use of fluorescently-labelled ligands in binding assays is the fluorescence quenching associated with receptors on cells or other large biomolecules. Fluorescence quenching can be due to a change in the conformation of the fluorescently labelled ligand, a change in its oligomeric state or the presence of nearby tryptophan, tyrosine, methionine, or histidine residues in the binding complex. This leads to a loss of correlation of the intensity of the fluorescent signal with the concentration of the ligand or ligand/biomolecule complex. In turn, this leads to a loss of sensitivity and accuracy of the assay itself.
Fluorescence polarization binding assays
Although fluorescence-based binding assays are in general more sensitive than absorbance, they can still suffer from background noise. This is especially true where cell lysates are being analysed for detection of biomarkers. The multitude of impurities within these samples can be problematic and purification steps still need to be performed. To lower this non-specific signal, light detection techniques have been developed, where the fluorescent signal does not directly come from detection of the fluorophore, but rather is moderated in some way to eliminate the background noise. Fluorescence polarization is one such technique.
Fluorescence polarization relies on the excitation of a fluorescent molecule with plane polarized light. When a fluorophore is excited with light there is a lag time (fluorescence lifetime) before it emits light of a longer wavelength. Small molecular weight molecules (<20,000 Da) labelled with fluorescein, can rotate in the assay buffer many times during their fluorescence lifetime. If polarized light is used to excite many of these molecules at once, as in an assay solution, then overall the light that is emitted is unpolarized, as the molecules are randomly orientated relative to each other when they emit. This effect can be used for binding assays when the small, labelled molecule is bound non-covalently to a much larger protein and the combined molecular weight is greater than 30,000 Da, the complex will not rotate very much at all during the fluorescence lifetime of the fluorophore (large molecules experience greater drag forces in solution). If a large molecular weight complex is excited with polarized light the light emitted will remain polarized (fig. 1). This property has been used to a great extent for binding assays in general. Only one of the binding partners needs to be labelled, which makes the assay relatively simple to setup and relatively cheap. Because you are only detecting polarized light, non-specific noise is greatly eliminated. One drawback is the molecular weight limit on the labelled binding partner. This needs to be below 30,000 Da (for fluorescein) and preferably much lower, otherwise the labelled molecule will emit polarized light irrespective of whether it is bound or unbound to another protein.
This Application Note on a Competition assay using Fluorescence Polarisation to determine the Residence Times for Calcitonin and AMYR agonist, AM833, demonstrates, that fluorescence polarization can be employed to determine association and dissociation rates of ligand receptor interactions.
Time-resolved fluorescence (TRF) binding assays
Another technique that has been developed to greatly eliminate background noise is time-resolved fluorescence (TRF). The strategy here is to use differences in fluorescence lifetime to separate the fluorescent signal of interest. Most fluorophores have a fluorescence lifetime of less than 10 ns. The lanthanides, Europium, Terbium, Dysprosium and Samarium, however, have very long lifetimes, ranging from 1 µs to 1 ms. In a typical TRF binding assay, one of the binding partners is labelled with a lanthanide, the sample is excited at the lanthanide excitation maximum (347 nm), and a time delay is introduced before detecting the signal (typically 400 µs). The time delay allows any background signal from fluorescent impurities in the sample to decay, leaving only the lanthanide fluorescent signal for detection. This again greatly improves the sensitivity over a simple fluorescence binding assay, with even less need for sample purification.
TRF has been used extensively in ELISA assays, especially via the DELFIA TRF assay system developed by PerkinElmer. This uses a europium label for detection. Many matched antibody pairs are commercially available that are specific to a biomarker of interest.
FRET – based binding assays
Foerster resonance energy transfer (FRET) is the radiation-less transfer of excitation energy from one fluorophore to another. Commonly, the fluorophore that is directly excited by light is termed the donor and the fluorophore excited by FRET is commonly called the acceptor. FRET can only occur between a pair of fluorophores when the distance between fluorophores is less than 10 nm. In addition, the emission spectrum of the donor must overlap with the excitation spectrum of the acceptor.
These properties of the FRET process have been utilised to great effect for binding assays in general (fig. 2). The two binding partners (proteins, DNA etc.) being studied, are each labelled with a fluorophore. If the two partners form a non-covalent interaction, then irradiation of the donor fluorophore will cause the acceptor to fluoresce as well, via FRET. In some ways, FRET can be thought of a fluorescent cascade (or domino effect) where one fluorophore excites the other, which then releases fluorescent light without direct excitation. As can be appreciated, the FRET signal given off by the acceptor fluorophore is very specific to the binding interaction being studied. FRET assays therefore are much more sensitive than simple fluorescence assays, this greatly decreases the need for purification steps in an assay and many assays are done in the presence of cell lysates. The main disadvantage of this method over fluorescence polarization, is the need to label both binding partners, which adds to the complexity and cost of setting up such a binding experiment. However, FRET-based binding assays are not limited by the molecular weight restrictions of fluorescence polarization.
The app note Using FRET-based Measurements of Protein Complexes to Determine Stoichiometry with the Job Plot shows how the assessment of protein-protein interaction stoichiometry has been successfully adapted to a microplate reader using a FRET-based approach.
Time-resolved FRET (TR-FRET)
TR-FRET binding assays can be thought of as modified FRET binding assays where the acceptor fluorophore is replaced with a lanthanide and are often used for studying signalling pathways and for screening agonists/antagonists of GPCR´s. In this talk, Prof. Charlton gives an overview, on how TR-FRET can be used to study and optimise binding kinetics.
The use of lanthanides allows both the introduction of a time delay before reading the assay and extra specificity in detection of the donor fluorophore via FRET. TR-FRET provides much greater sensitivity in a binding assay than either method alone, due to decreased background fluorescence. This increased sensitivity, allows a good LOD with much smaller volumes of reagents, a signal can be detected in as little as 2 µl total volume, with minimal purification of analytes required. This allows the use of 384-, 1536- and 3456-well microplates making TR-FRET a method of choice for high-throughput screening (HTS) binding assays. There are three major chemistries that when combined with appropriate antibodies (and other affinity tags such as biotin), allow detection of a huge number of binding interactions; Homogeneous Time Resolved Fluorescence (HTRF®), LanthaScreen™ and Lance®. The donor and acceptor characteristics for the three are detailed in table 1. All these chemistries are available in kits for screening for specific biomarkers, and various binding partners and there are strategies available for labelling new and novel binding partners.
Table 1: Donor and acceptor characteristics of TR-FRET technologies.
TR-FRET Chemistries | Donor | Ex Max | Acceptor | Lanthanide Em Max | FRET Em Max |
Lance | Eu-Chelate | 347nm | APC | 620nm | 665 |
HTRF | Eu, Tb Cryptate | 347nm | XL665(APC), d2 | 620nm, 490nm | 665 |
LanthaScreen | Tb-Chelate | 347nm | Fluorescein | 490nm | 520 |
Besides the use of commercially available kits, TR-FRET donor and acceptor pairs can also be combined freely, as long as the donor emission spectrum shows sufficient overlap with the acceptor’s excitation spectrum., as shown in AN 388: Differential binding of ∆9-tetrahydrocannabinol derivatives to type 1 cannabinoid receptors (CB1).
All TR-FRET experiments have two emissions, one via FRET excitation of the fluorophore and one emission from the lanthanide. Most TR-FRET experiments calculate the FRET ratio (signal of fluorophore/signal of lanthanide) rather than just the FRET signal, this is the case in fig. 14b. Calculation of the FRET ratio (665 nm/620 nm, in this case) eliminates possible media interferences and means the assay is unaffected by the usual experimental conditions (e.g. culture medium, serum, biotin, coloured compounds).
In the scientific talk Targeting the type 1 cholecystokinin receptor to screen for novel obesity treatments it is discussed how a TR-FRET binding assay was established to filter out orthosteric or negative-allosteric binders.
There are also examples of TR-FRET competitive binding assays which read two signals at the same time (multiplexing). These assays typically use a Terbium-labelled donor, and a green and a red dye as the acceptors.2
BRET (bioluminescent resonance energy transfer) binding assays
Bioluminescence has been used extensively in biological research for some 40 years. Luminescence assays use luciferase enzymes to generate light. The first luciferase to be isolated and cloned in bacteria was the firefly luciferase, from the North American firefly.3 Since then, many luciferases have been identified. Luciferases react with their natural substrates (luciferin, for the American firefly) and in the presence of ATP and oxygen produce an oxidation product (oxyluciferin, for the American firefly). The product is formed in an excited state, light is produced when the excited electron relaxes to a lower energy level.
In the last 20 years, luciferases have increasingly also been used for BRET binding assays. BRET assays are very similar to FRET and TR-FRET assays, except that the donor used for one of the binding partners is a luciferase. The incorporation of a luciferase, as the acceptor, allows direct expression of a protein of interest (such as a GPCR) with the luciferase label and this can be used in cell-based binding assays relatively easily as compared to FRET and TR-FRET experiments. The other important advantage of BRET assays is that no light is needed for stimulation, the only requirement is addition of a luciferase substrate. This alleviates complications such as fluorophore bleaching, and unwanted generation of background fluorescent signals from other cellular components, when using a light source to excite your fluorophore or lanthanide. The first BRET assay was first reported in 1999, since then many luciferases have been tried as donors as well as many fluorophores as acceptors. The most important advance was the engineering of the NanoLuc luciferase, a small molecular weight enzyme that has a luminescence output 150x greater than Firefly. The NanoLuc luciferase has been developed into a BRET platform called NanoBRET™ by Promega, for the study of protein-protein interactions in live cells.
One important application of BRET is the study of the binding of agonists to their receptors on the membranes of cells in real time. This is also further discussed in the webinar: “Real-time profiling of receptor pharmacology”.
AlphaScreen®-based binding assays
The AlphaScreen® (amplified luminescent proximity homogeneous assay) light stimulation platform for binding assays has been available commercially for around 20 years. The heart of the assays consists of two distinct types of polymer beads. The donor bead contains a photosensitiser within its structure, which when irradiated with 680 nm light generates singlet oxygen in the surrounding aqueous media. The acceptor bead incorporates a chemiluminescent compound within its structure. Singlet oxygen has a lifetime of ~4 µs and travels about 200 nm in solution during this time. If the donor bead is in close-proximity to the acceptor bead, then the acceptor will produce light via luminescence at wavelengths of 520-620nm. The beads can be used to label various proteins of interest, via antibodies (specific to the targets) that are attached directly to the beads. Fluorescence always proceeds by emission of light at a longer wavelength than which the fluorophore is excited. AlphaScreen works in the reverse direction, therefore any background fluorescent signal from the 680nm stimulation is not detected using this method. This generally makes the technology very sensitive.
A typical example of a ligand binding assay employing AlphaScreen technology, is the development of a competitive binding assay for studying the interaction of the protein ENL-Yeats domain binding to Histone H3. The ENL-Yeats protein complex binds via the Yeats peptide to Histone H3 when several lysine residues on the histone are either acetylated or crotonylated at the lysine side chain amino group. The histone interaction with Yeats-ENL has been shown to be a major driver leading to several types of acute leukaemia.
One problem with the AlphaScreen technology is that AlphaScreen beads are light sensitive and need to be prepared in low light conditions. The signal decays slowly over time and microplates need to be read very soon after they are prepared. The signal does not last if plates are stored in a refrigerator overnight, in contrast to TR-FRET based mixtures which last much longer when prepared in a microplate. Although background fluorescence is removed, antioxidants and metal ions that can react with singlet oxygen can strongly affect the signal. To counteract these drawbacks AlphaLisa binding assays were developed, which employ acceptor beads that are infused with Europium resulting in greater sensitivity and reliability.
As is evident from this article, there are many ways to perform binding assays. Which one to choose will be determined in part, by the plate reader that is available, the cost of various kits or the cost of labelling the binding partners for a particular assay.
Plate readers come in various configurations from low budget models to high-end units capable of reading any of the assays described here, with high sensitivity. However, most models, apart from absorbance only microplate readers can read as a minimum fluorescence, luminescence, and absorbance. If this is the case, then fluorescence-based binding assays would be preferred over absorbance-based ones as they give a more intense and specific signal over a much wider range. In the case of ELISA assays less wash steps are involved in a fluorescence-based assay. In fact, nearly all absorbance assay kits on the market today have a fluorescence-based equivalent including enzyme assays, protein concentration assays, cell viability assays etc. The fluorescence-based kits are slightly more expensive but are simpler and more sensitive to use. High-end readers, compatible with further detection techniques, offer an even greater choice of kits for performing binding assays, including FRET, TR-FRET or AlphaLisa-based assays. These combine the advantages of an easy setup with greater sensitivity.
A further consideration is that no one technique is perfect. The light signal from each technique can be affected in an unpredictable manner in the presence of individual compounds in a library, in cells or bodily fluids. In high-throughput screening of compound libraries, it is common practice to develop an assay based on a few techniques, as actives identified in one assay do not totally overlap with those identified in another. To give an example, in one study, two TR-FRET (Lance, HTRF) and one AlphaScreen assay were developed to screen 90,000 compounds, for inhibitors of SIRPα-CD47 binding. In a pilot screen, the three assay technologies displayed varying activity rates (0.2% for Lance and 1.1% for HTRF and AlphaScreen) with some overlap. The authors found that the Lance assay gave less false positives than HTRF and used Lance for the primary screen and AlphaScreen as an orthogonal confirmation assay.5 It is thus crucial to test various methods and determine the best suited for performing binding assays for your target of interest.
In conclusion, of the multitude of binding assays found in the literature, most can be categorised according to the light detection technology being used. Likewise, most of the light detection techniques can be used to develop a binding assay and it is crucial to select a suitable microplate reader. The CLARIOstar® Plus and the PHERAstar® FSX are BMG LABTECH´s go-to readers for performing binding assays and are compatible with all common detection technologies. While the CLARIOstar Plus offers maximal flexibility in binding assay performance due to the LVF and EDR technologies, the PHERAstar FSX combines highest sensitivity and speed due to the SDE feature and a laser-based light source.
Moreover, the AAS system provides a steady temperature that is unaffected by external environmental changes while the plate reader is operating. This provides improved assay stability for temperature-sensitive reactions such as binding reactions.
Hopefully, the information contained in this article will help to make a better-informed choice for selecting a suitable binding assay. For more information and examples, visit our website or contact us at applications@bmglabtech.com.
Absorbance plate reader with cuvette port
Powerful and most sensitive HTS plate reader
Most flexible Plate Reader for Assay Development
Upgradeable single and multi-mode microplate reader series
Flexible microplate reader with simplified workflows
Innovation for targeted protein degradation and next-generation degraders is gathering pace. This blog introduces some of the different approaches that act via the lysosome or proteasome.
The choice of assay for targeted protein degradation studies is crucial. But what is the preferred assay and detection technology for your specific research needs and microplate reader?
Molecular glues are small molecules that help target unwanted proteins for destruction by the ubiquitin-proteasome system. Find out how microplate readers can advance molecular glue research.
Cannabinoids offer exciting opportunities to target diverse diseases with unmet needs. Learn how microplate readers can help improve our understanding of drug screening and drug signaling events to help advance cannabinoid research.
PROTACs are small, readily designed molecules that target unwanted proteins to the cell’s ubiquitin-proteasome system for degradation. Find out how microplate readers can advance PROTAC research.
Measuring light scattering is an accurate and expedient way to determine drug solubility. Find out how the NEPHELOstar® Plus can be used for early drug solubility screening at high throughput.