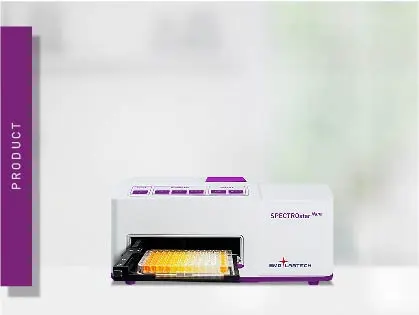
SPECTROstar Nano
Absorbance plate reader with cuvette port
Researchers have different technology options available for absorbance measurements. This blog compares spectrometers and monochromators. What’s the difference?
The fundamental observation that almost all molecules in nature absorb and emit light at different wavelengths has driven developments in spectroscopy and fueled discoveries across scientific disciplines. 1 Today researchers have access to technologies and instruments that use different forms of light to generate precise measurements of how much of a particular substance is present in a solution. These technologies are also useful in identifying molecules, analyzing the composition of samples, and studying molecular properties and processes. Light can be readily used to quantify the concentrations of substances such as nucleic acids, proteins and other molecules. It can also be deployed to measure the activities of enzymes or assess the levels of contamination in biological samples.
But what are the technologies behind the applications and what are the preferred options for making absorbance measurements in microplates? In this blog we look at monochromators and spectrometers, two different ways of manipulating light to enable biological measurements, and their uses for absorbance measurements. We also look at some widely used absorbance applications where a spectrometer gives the user an extra edge in time and efficiency.
A monochromator is an optical device that produces a narrow band of wavelengths from a broader spectrum of light. Specific wavelengths are selected and transmitted through a monochromator while others are blocked. In contrast, a spectrometer allows the measurement of light intensity throughout the entire ultraviolet and visible parts of the spectrum (Fig. 1).In practical terms, a monochromator only captures one measurement in the ultraviolet-visible spectrum at a particular wavelength or a specific bandwidth and therefore only gives one value. On the other hand, a spectrometer captures the entire spectrum in the same amount of time and generates values at every wavelength.1
Monochromators have been an important technology in microplate readers for years and are an integral part of spectrophotometers. Monochromators essentially come in one of three main types: prisms, diffraction gratings or filters. Prisms offer good wavelength separation but can be subject to nonlinear dispersion and may not cover a wide spectral range. Diffraction gratings offer high resolution, linear dispersion, and can cover a broad range of wavelengths. Filters provide simple and cost-effective wavelength selection but have a limited spectral range and lower resolution compared to prisms and diffraction gratings.
The main components of monochromators to separate different wavelengths of light from a given light source are typically an entrance slit, mirrors and the light disperser. The different options for the disperser of light include one of the three main types of monochromators mentioned earlier: the prism, grating or optical filter. Lenses and mirrors direct the dispersed light towards the exit slit. The exit slit selects the narrow band of wavelengths and allows it to leave the monochromator. Focusing optics may be used to direct the selected band of wavelengths onto a detector or other target.
The essential components of monochromators are similar but there are different ways to set them up. Mirrors can be made from materials like aluminum, silver or gold that can be coated onto glass or other stable substances that will allow them to reflect light within the monochromator. Slits are typically made of metal that is precisely machined to provide an opening that controls the light beam entering and exiting the monochromator. Lenses constructed from optical-grade glass, quartz or plastic, focus and direct the light to where it is needed. Optical coatings are often applied to components of the monochromator to reduce or enhance reflection of light as needed. In advanced monochromators, motorized controls provide further precision and assist in the optimal selection of wavelengths.
The most widely found arrangement of components in a monochromator is referred to as the Czerny-Turner arrangement which comprises two concave mirrors and a diffraction grating.2 Light rays pass through the slit and travel to the first mirror which arranges the rays of light so that they travel in parallel to one another. The parallel rays strike the diffraction grating which bends the different wavelengths of light at different angles. The next destination is the second concave mirror which focuses the selected light. The grating, a crucial part of the monochromator, may be rotated to control the range of wavelengths passing through the device. The end destination is typically a charge coupled device or CCD detector.
Today’s optical instruments for absorbance measurements typically contain more than one monochromator. While a double monochromator decreases the intensity of light reaching the detector it helps to improve the resolution and accuracy of measurements. The passage of light through the two monochromators helps to remove undesirable wavelengths or stray light that can interfere with the accuracy of the final measurements.
As mentioned earlier, at the heart of most monochromators is a diffraction grating made of glass or metal on which there are a series of regularly spaced parallel slits, ridges or rulings. The ridges and rulings generate grooves on the surface of the grating. Light hits the grating and its grooves and depending on its wavelength is diffracted at different angles. Several gratings may be included in a monochromator. In gratings used for probing the infrared range of the light spectrum, the spacing between the grooves on the surface of the grating is wider. Gratings used for the ultraviolet and visible range (typical for absorbance measurements) have much tighter spacings between grooves.
Imperfections on the surfaces of gratings limit their quality. Resolution improves when more grooves are present on the surface. Decreasing the spacing between grooves will increase the dispersion of incident light and resolution of the different wavelengths. Monochromators are most frequently employed for ultraviolet-visible absorption measurements that have multiple applications. They provide an effective way to study a wide range of sample types including for example proteins, drugs, pharmaceuticals, DNA and RNA. These types of analysis are often performed in a microplate reader.
Monochromators are effective tools for looking at changes in absorbance at specific wavelengths . A monochromator can also be used to scan through an entire wavelength range for each measurement. However, while a monochromator can be used to acquire a broader spectrum the scanning is fairly slow, and a full scan may take around one minute or more. In contrast, a spectrometer instantly captures an entire spectrum of measurements offering much greater speed. Let’s take a closer look at a spectrometer.
The strengths of a spectrometer for absorbance analysis lie in its ability to capture the whole ultraviolet-visible spectrum (220-1000 nm) of a sample in a very short time without the need for scanning. A spectrometer incorporates a highly efficient optical grating and a solid-state array detector that allows the measurement of light intensity throughout the entire ultraviolet and visible parts of the spectrum (Fig. 1). The spectrometer disperses light into its component wavelengths and measures the intensity of each wavelength.
The key components of a spectrometer include a light source (typically tungsten, deuterium or a xenon flashlamp), an entrance slit to control the size of the light beam, a dispersive element (like a monochromator this can either be a prism or more likely a diffraction grating), lenses and mirrors to direct the dispersed light toward the detector, and a detector which may either be a photomultiplier tube (PMT), CCD or photodiodes. At the structural level, a spectrometer uses a detector array to measure a broad range of wavelengths simultaneously while a monochromator uses slits and a single point detector to isolate and measure a narrow band of wavelengths one at a time.
The light from the light source (tungsten, deuterium or a xenon flashlamp) enters the spectrometer through an entrance slit which narrows the beam but keeps the broad spectrum intact. This helps to improve the resolution and directs the beam accurately onto the dispersive element. A diffraction grating is most often used as the dispersive element separating the light into its component wavelengths. Lenses or mirrors are used to generate parallel rays of light of different wavelengths that are directed towards the detector. The detector, which can be a CCD or diode array, measures the intensity of light at each wavelength. The detected signal is converted into an electrical signal which is processed and digitized. The result is a spectrum plotted as light intensity versus wavelength (Fig. 2).
What are the benefits of a spectrometer over a conventional monochromator? The biggest advantage is that unlike conventional monochromators, an ultraviolet/visible absorbance spectrometer will instantly capture an entire spectrum much more quickly with no scanning needed. The user does not have to worry about setting up or tuning different wavelengths for absorbance assays.
The ultraviolet/visible (UV/vis) spectrometer from BMG LABTECH instantly captures an entire spectrum from 220 to 1000 nm with no scanning needed. The spectrometer will capture full-absorbance data with an adjustable resolution of up to 1 nm for all wavelengths in the 220 to 1000 nm range in less than 1 second/well. Alternatively, up to eight discrete wavelengths can be collected at the same time.
The capabilities of the spectrometer address a wide range of applications. Time course experiments can be monitored for wavelength shifts with a resolution of <0.3 seconds due to the ultra-fast speed of spectral capture. Absorbance shifts can be monitored in real time as an assay goes to completion.
Spectrometers for microplates offer many solutions for everyday challenges in the life sciences. From protein determination or assessing the purity of DNA or RNA samples to redox measurements for enzyme reactions and enzyme-linked immunosorbent assays (ELISAs), ultraviolet-visible spectrometers are adept at reliable and fast measurements at high sensitivity for diverse biological samples.
In the application note Absorbance-based methods for protein quantification several examples are presented for the standard absorbance-based protein quantification assays that are readily performed in the laboratory. BMG LABTECH spectrometer-based readers capture absorbance spectra or multiple absorbance measurements at discrete wavelengths from 220-1000 nm in less than a second per well and therefore can quickly and efficiently read assays even on full microplates. Some of the commonly used methods such as measuring the absorbance at 280 nm, the Bradford assay (Fig.3)3, the Lowry assay4, and bicinchoninic acid (BCA)5 method are presented. Predefined protocols support easy and quick measurements. One-click calculations of protein concentrations are available using the MARS analysis software.Assessing the purity of nucleic acid samples is another frequently performed application in the laboratory. The quantification of nucleic acids (DNA and RNA) is a central step in molecular biology as downstream experiments rely on the use of adequate amounts of nucleic acids. In addition to the quantification of nucleic acids, the determination of the DNA purity ratio is crucial since impurities or contaminants may affect the successful implementation of subsequent experiments using the purified DNA samples (Fig. 4). An example of how the ultraviolet-visible spectrometer capability enables fast and easy, simultaneous detection of multiple wavelengths is demonstrated in the application note DNA purity ratio: evaluation of nucleic acid quality. Absorbance readouts allow the detection of multiple wavelengths for all-round DNA quality assessment. Compared to the monochromator-based acquisition of the four relevant wavelengths, which takes about 10 s/well with a competing instrument, a significant time saving can be achieved with the spectrometer. This approach allows fast and easy nucleic acid quality assessment with the simultaneous recording of multiple wavelengths.
In the application note ELISA assays and NADH/NADPH conversion detection the benefits of using the spectrometer for measuring enzyme-linked immunosorbent assays (ELISAs) or assays that need the cofactors NAD+, NADH, NADP+ and NADPH are demonstrated.
ELISAs are an essential technique in today’s laboratory with many applications in the life sciences.6 They are often the method of choice to detect or measure specific biological molecules (analytes) for diagnostics, drug discovery or fundamental research. The spectrometer gives researchers the flexibility to select either a full spectrum (220-1000 nm) or part of a spectrum (350-530 nm) for their ELISA measurements and enables rapid and sensitive measurements as needed at the optimal wavelengths (Fig. 6).
NADH/NAD+ and NADPH/NADP+ are cofactors used by many enzymes including those involved in energy metabolism, mitochondrial function, calcium homeostasis, oxidative stress, gene expression, immunological functions, aging and cell death. The reduction of NAD+ to NADH and NADP+ to NADPH can be monitored at 340 nm because the oxidized forms do not absorb light at this wavelength (Fig. 7). By using the spectrometer feature researchers can select either a full spectrum (220-1000 nm) or part of a spectrum (220-400 nm) to achieve rapid and accurate measurements of redox states.
The demand for accuracy and speed of absorbance measurements is greatly facilitated using a ultraviolet-visible spectrometer over conventional monochromators. Absorbance measurements are widely used in essential applications across the life sciences. The choice of a spectrometer in a microplate reader will continue to offer distinct advantages to researchers looking to carry out their absorbance detection assays with efficiency and rigor.
Absorbance detection with the signature ultra-fast UV/vis spectrometer is available on BMG LABTECH’s single- and multi-mode microplate readers. The exception is the NEPHELOstar® Plus which is a dedicated laser-based nephelometer for light scattering and turbidity measurements.
BMG LABTECH was the first plate reader manufacturer to incorporate a UV/vis spectrometer for absorbance detection into its multi-mode instruments. Unlike conventional monochromators, BMG LABTECH’s ultrafast spectrometer instantly captures an entire spectrum from 220 to 1000 nm in less than 1 second/well with no scanning needed.
BMG LABTECH also offers a range of multi-mode detection devices for sensitive fluorescence and luminescence measurements. Furthermore, the VANTAstar®, the CLARIOstar® Plus, the Omega series and NEPHELOstar® Plus can be combined with the Atmospheric Control Unit making them the preferred choice for different kinds of live cell assays including absorbance-based OD600 bacterial growth assays.
Both the VANTAstar and CLARIOstar Plus further allow for wavelength flexibility and include Enhanced Dynamic Range technology for superior performance in a single luminescence or fluorescence run. They also offer increased light transmission and sensitivity courtesy of Linear Variable Filter MonochromatorsTM and different filter options.
All BMG LABTECH microplate readers have exceptionally fast reading capabilities. In addition, the Omega series, CLARIOstar Plus, and PHERAstar® FSX microplate readers come with on-board injectors that can offer the very best options for detection at the time of injection. The VANTAstar can be equipped with a modular injection unit for this purpose.
Collectively, BMG LABTECH multi-mode readers combine high-quality measurements with miniaturised assays, short measurement times, and offer considerable savings on materials and other resources.
Absorbance plate reader with cuvette port
Powerful and most sensitive HTS plate reader
Most flexible Plate Reader for Assay Development
Upgradeable single and multi-mode microplate reader series
Flexible microplate reader with simplified workflows
The Z prime value (Z’) is a statistical parameter that can provide practicable information on the quality of an assay. This blog looks at its usage and describes some examples of specific applications.
Optical density and absorbance measurements are widely used in the life sciences. This blog looks at practical applications and some of the fundamentals.
ELISAs are a popular tool to detect or measure biological molecules in the life sciences. Find out how microplate readers can be used to advance research using immunoassays.
LanthaScreen is a TR-FRET technology which can be used to measure kinase activity, compound binding, and post-translation modification events. Read more about this type of assay here.
Although the concept of fluorescence gain has always been hard to grasp, it is a make-or-break parameter in microplate reading. Read here how to adjust it optimally.