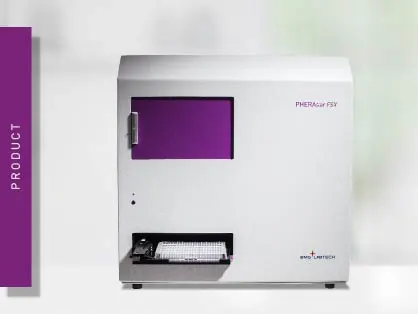
PHERAstar FSX
Powerful and most sensitive HTS plate reader
Many biological processes are driven by the interaction of two molecules. Enzymatic reactions, protein-protein binding, DNA replication, or receptor-ligand interactions are just a few examples that inevitably depend on the close proximity of two binding partners and their binding affinity to each other. To induce their effect, drugs are often specifically designed to target molecular interactions associated with specific diseases. Accordingly, in the fields of pharmaceutical research, and drug discovery, the determination and optimisation of binding affinity are of great importance for the development of new drugs and treatment options.
Binding affinity is the strength of binding interaction between a biomolecule and its ligand or binding partner. This intermolecular interaction is often facilitated by non-covalent means such as hydrogen bonding, electrostatic interactions, hydrophobic and Van der Waals forces.
Thus, the compatibility of binding partners is not merely restricted to a single class of biomolecules but rather dependent on their overall molecular and three-dimensional structures. This way binding can be achieved between a multitude of biomolecules such as proteins, nucleic acids, hormones or second messengers.
Binding affinity plays an important role in basic research, as well as in modern medicine and drug discovery 1,2,3. Due to its function as a determining factor in many biologic interactions, it is often investigated in related research. From the determination of the binding characteristics of antibodies, nucleic acids or proteins, the characterisation of substrates and inhibitors to the discovery of novel endogenous interaction partners, binding affinity has many uses.
In drug research, it is used as a measure of the quality of a drug candidate. Knowledge of the affinity of a potential drug to its target structure makes it possible to draw conclusions on its selectivity, potency, and optimal dosage. Generally speaking, the higher the affinity of a drug to its target, the better, since lower amounts of the drug are sufficient to induce the desired effect, reducing the risk of adverse side effects.
Typically, binding affinity is represented by the equilibrium dissociation constant KD, the concentration of ligand at which half the binding sites on a biomolecule are occupied at equilibrium. The KD value describes the propensity of a complex (BL) to dissociate into its original components (B and L), in this case, the biomolecule and its binding partner. The larger the binding affinity of a ligand to its target, the smaller its propensity to separate from it. Accordingly, a low KD value is associated with a high binding affinity and vice versa. Since binding is not static and changes over time, binding affinity is determined in so-called binding kinetics.
Calculation of binding affinity based on the rate constants kon and koff
Like its inverse, the association constant KA, KD can be calculated using the rate constants kon and koff. In the equilibrium reaction of complex formation and dissociation, kon is the rate at which the complex BL is formed out of biomolecule B and ligand L. As such, it is dependent on the concentrations of B and L, as well as time, and is measured per molar per second (M-1 s-1). Conversely, koff, the rate at which complex BL dissociates into B and L is concentration-independent and measured per second (s-1).
The dissociation constant KD can be calculated by dividing koff by kon. As it represents a ligand concentration, it is measured in molarity (M). Alternatively, KD values can also be calculated from the concentrations of biomolecule B, ligand L and complex BL at equilibrium.
Graphical determination of the binding affinity based on saturation curves
Apart from mathematic calculations, KD can be determined by non-linear regression from saturation curves. Since it represents the concentration of ligand at which half the ligand-binding sites of a biomolecule are occupied at equilibrium, KD values can be derived from saturation binding graphs by plotting the percentage of occupied biomolecule over the ligand concentration (fig. 1).
Determination of binding affinity based on competition binding assays
Alternatively, KD values can be determined by competition binding assays. Here, a potential untagged ligand is added in increasing concentrations to its target biomolecule which was treated with a known and tagged reagent. Dependent on its binding affinity to the target biomolecule, the untagged ligand of interest displaces the tagged reagent (competition) which results in a signal decrease. Employing the Cheng-Prusoff equation, the KD value of the untagged ligand of interest can be calculated from its IC50 value. 4
Microplate readers are an essential tool in binding affinity related research. With smaller sample volumes, short evaluation times and the ability to investigate multiple conditions in one test run, the microplate-based assay format supports upscaling processes, which enable the determination of binding affinity and the identification of interaction partners and potential drugs in high-throughput. Additionally, microplate readers deliver robust and reproducible results and can easily be integrated into automated systems, which makes them ideal platforms for integration in high-throughput facilities.
There are a plethora of binding assays which can be employed in the investigation of binding affinity. The most relevant microplate-based measurement techniques utilised to determine binding affinity are described below. These employ the full range of detection modes, from absorbance, AlphaScreen®, fluorescence intensity, time-resolved fluorescence (incl. TR-FRET) and fluorescence polarization to luminescence.
FRET assays
FRET (Förster´s resonance energy transfer) is a detection technology that shows the energy transfer between two fluorophores, one donor and one acceptor, attached to two interaction partners of interest and thereby allows to study of molecular interaction. Most commonly, genetically encoded fluorescent proteins such as green, red, or yellow fluorescent protein are used as so-called “FRET pairs” to provide donor and acceptor fluorophores.
FRET is named after the German scientist Theodor Förster who originally developed the theory of resonance energy transfer 5. For it to take place, the donor fluorophore that has absorbed energy upon external excitation and finds itself in an electronically excited state has to transfer energy to the acceptor fluorophore, which can only happen if it is in close proximity (fig. 2). The transfer of energy reduces the emission intensity of the donor while increasing the acceptor’s emission intensity which serves as readouts of the assay. Accordingly, two separate emission channels are needed to monitor donor and acceptor emission simultaneously.
Since the acceptor fluorophore only emits a signal if the binding between the two interaction partners of interest occurs, it can be used to investigate their interaction and binding affinity. In this context, FRET assay systems are often employed by the pharmaceutical industry to screen for new drug candidates.
Generally, a competitive setup is used to avoid the need to label large numbers of potential inhibitors prior to screening. Here, instead of the potential inhibitors, the corresponding target structures are labelled with fluorophores: a target bearing the donor and a known target binder bearing the acceptor molecule. If a compound displaces the acceptor-labelled molecule, the acceptor signal decreases due to separation, which signals binding inhibition. Binding affinity can then be determined from the ratio of acceptor to donor emission. This way, large compound libraries can be screened for binding affinity to a target biomolecule in a fast and efficient manner.
TR-FRET
Time-resolved FRET (TR-FRET) improves the FRET detection technology by introducing time-resolved fluorescence (TRF) and time-delayed detection. This method eliminates the short-lived background noise which can derive from scattered excitation light and autofluorescence by employing lanthanides as donor molecules.
These long-lived fluorophores have large Stokes shifts and emission lifetimes up to milliseconds in length (compared to microseconds of classical fluorophores), enabling the application of a time delay between excitation and fluorescence detection in the microsecond range. This way, buffer or medium interference is significantly reduced by the long-lived fluorescence emission of the lanthanides and by the ratiometric detection of the two emission wavelengths of donor and acceptor (fig. 3). Because of these properties, TR-FRET offers improved stability and specificity over standard FRET and can easily be adapted for cell-based assays and/or high-throughput screenings.
The general principle of how this technology is applied, is very similar throughout the different kit manufacturers, the most popular of which include HTRF®, LANCE® and LanthaScreenTM. Donor and acceptor are either covalently bound to the interacting partners or a specific antibody against each of the two targets (or tags) is labelled with either the donor or the acceptor.
The major advantage of this method is that detection of the intramolecular interaction does not require physical separation from the unbound components since background signals are reduced by time-delayed detection. As so-called homogeneous assays, TR-FRET assays do not need in-between washing steps and can be run as simple add-and-read assays, minimising handling steps and being more convenient and less time-consuming than ELISAs. Accordingly, TR-FRET can easily be adapted for high-throughput analysis of protein aggregation or protein-protein interactions. For further information see also: AN 286: Detection of human tau protein aggregation, AN 335: Analyze binding kinetics with HTRF and AN 388: Differential binding of ∆9-tetrahydrocannabinol derivatives to type 1 cannabinoid receptors (CB1).
Fluorescence polarization assays
Fluorescence polarization (FP) is a fluorescence-based detection method that is widely used to investigate molecular interactions and binding affinity in solution. However, in contrast to fluorescence intensity, FP does not solely focus on the emission wavelength of a fluorophore upon excitation but also on the analysis of the polarization of the emitted light.
As an electromagnetic wave, the electric field of light oscillates perpendicularly to the direction of propagation, determining its polarization. In the case of unpolarized light, the direction of this oscillation fluctuates randomly in time. By employing specific filters oscillation can be selected, so that all selected light waves will oscillate in a single plane, producing plane-polarized light (fig. 4).
FP can be used to analyse molecular interactions and to determine binding affinity by performing binding kinetics. Alternatively, dissociation and enzymatic degradation assays can be measured with FP, utilising the decrease in molecular volume and subsequent increase in depolarized light as readout.
Using this principle, the interaction of transcription factor p53 with different DNA sequences was quantified by employing p53, fluorescently labelled DNA and competitor DNA sequences without an attached fluorophore. In titration experiments, the competitor DNA displaced the labelled DNA from p53 and the subsequent change in FP of labelled DNA was used to calculate the dissociation constant KD. You will find more information on this topic in AN 180: High-throughput protein DNA measurement using fluorescence anisotropy. See also AN 256: Protein-ligand binding measurements using fluorescence polarization.
Fluorescence polarization is also well suited to study binding kinetics during ligand receptor interaction. In contrast to classically used assays based on (TR-)FRET- or BRET, FP-based assays do not require additional labelling of the receptor next to the ligand. This application note shows the successful application of a Competition assay using Fluorescence Polarisation to determine the Residence Times for Calcitonin and AMYR agonist, AM833.
BRET assays
BRET (bioluminescence resonance energy transfer) assays are luminescence-based methods that can be used to determine binding affinity and are often employed to investigate protein-protein interactions. Like FRET, they are based on resonance energy transfer, but in the case of BRET, the donor energy is generated by bioluminescence with one interaction partner coupled to luciferase and the other to an acceptor fluorophore. Upon interaction of both partners, the luminescence energy produced by the luciferase is transferred to the acceptor molecule and the acceptor emission can be measured with a luminescence plate reader or with a multi-mode plate reader (fig. 6 A).
The binding affinity of a ligand to its interaction partner can be determined by saturation or competition binding assays from the raw BRET ratios of acceptor to donor emission (fig. 6 B). For this purpose, researchers at Promega developed a genetically engineered luciferase called NanoLuc® (Nluc), which is ideally suited to act as BRET donor due to its small size and high stability 6. See also: AN 287: NanoBRET assay for monitoring of ligand binding to GPCRs in live cells, using the CLARIOstar and the PHERAstar FS. Novel approaches using the NanoBIT® system, which employs a fragmented nanoluciferase attached to two binding partners of interest, are frequently used nowadays. Upon binding, the nanoluciferase fragments form a functional enzyme that can generate a luminescence signal as highlighted in the application note: Identification of androgen-disruptors using a cell-based androgen receptor dimerization assay.
AlphaScreen assays
AlphaScreen® (ALPHA for Amplified Luminescent Proximity Homogenous Assay) uses bead-based chemistry to study intermolecular interactions. For this purpose, ligand and target biomolecule are labelled with either of two types of hydrogel-coated beads, called the donor or acceptor beads.
AlphaScreen donor beads contain a photosensitizer that converts oxygen (O2) into excited singlet oxygen (1O2) upon excitation at 680 nm. In turn, 1O2 reacts with chemical dyes in the acceptor beads. The transfer of chemical energy results in broad light emission from 520 nm to 620 nm which can be measured with a microplate reader.
Due to the short half-life time of excited 1O2 (4 µs), energy transfer and chemiluminescence can only occur if both types of beads are in close proximity to each other. Accordingly, AlphaScreen assays are perfectly suited to monitor the interaction of potential binding partners.
AlphaScreen technology, which includes AlphaScreen, AlphaLISA® and AlphaPlex™ assays, is mainly used in high-throughput screening assays to assess biomolecular interactions due to its very high sensitivity. Employing this principle, Christott et al. used an AlphaScreen assay to screen for selective inhibitors of the human YEATS domain and determined their binding affinity by analysing dose-response curves and IC50 values. For more information see AN 320: PHERAstar measures AlphaScreen assay to develop selective inhibitors for the human YEATS domains.
Absorbance-based methods
While methods based on AlphaScreen, BRET, FP or FRET are used most often in binding studies, there are also some assays that utilize absorbance as a readout to investigate molecular interaction and binding affinity. These are based on the quantification of an absorbance signal or its change over time.
For example, competitive ELISA assays can be employed to determine the binding affinity of antibody pairs. However, the often-heterogeneous principle of this kind of assays limits their application in an automated high-throughput setting, since additional washing and handling steps are needed in comparison to homogeneous BRET, FRET, TR-FRET or FP applications.
Modern microplate readers offer several advantages for the determination of binding affinity and interaction assays such as increased performance and sensitivity. They deliver robust results, coupled with minimal variability and larger assay windows. Features such as Simultaneous Dual Emission, Decay Curve Monitoring, Enhanced Dynamic Range and the improved detection of fluorophores in the red range help to further reduce background signals and measurement time while improving sensitivity.
Furthermore, microplate readers are the perfect platform for the high-throughput screening of molecular interactions, protein-protein binding, and drug interactions. Homogenous interaction assays, such as AlphaScreen, BRET, FP, FRET and TR-FRET based methods, do not require washing steps to remove unbound components from your assay and are based on a mix and read principle. This can minimise both, handling steps and operation time, which promotes them particularly in automation-supported screening and in the field of drug discovery.
Do you have any additional questions in regard to binding affinity applications? Feel free to contact the BMG LABTECH application support at applications@bmglabtech.com for additional information on this matter.
Powerful and most sensitive HTS plate reader
Most flexible Plate Reader for Assay Development
Flexible microplate reader with simplified workflows
Innovation for targeted protein degradation and next-generation degraders is gathering pace. This blog introduces some of the different approaches that act via the lysosome or proteasome.
The choice of assay for targeted protein degradation studies is crucial. But what is the preferred assay and detection technology for your specific research needs and microplate reader?
Molecular glues are small molecules that help target unwanted proteins for destruction by the ubiquitin-proteasome system. Find out how microplate readers can advance molecular glue research.
Cannabinoids offer exciting opportunities to target diverse diseases with unmet needs. Learn how microplate readers can help improve our understanding of drug screening and drug signaling events to help advance cannabinoid research.
PROTACs are small, readily designed molecules that target unwanted proteins to the cell’s ubiquitin-proteasome system for degradation. Find out how microplate readers can advance PROTAC research.
Measuring light scattering is an accurate and expedient way to determine drug solubility. Find out how the NEPHELOstar® Plus can be used for early drug solubility screening at high throughput.