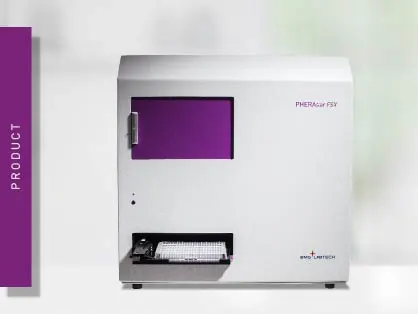
PHERAstar FSX
Powerful and most sensitive HTS plate reader
Mitochondrial toxicity can have devastating effects on the cell and life. Find out how microplate readers can be used to assess mitochondrial health and how this impacts disease and drug discovery.
Around 1.5 billion years ago an ancient cell engulfed a bacterium to form what we know today as the eukaryotic cell (fig. 1). The engulfed bacterium evolved into the mitochondrion, a double-membrane-bound organelle often called the “energy factory” or “powerhouse” of the modern cell.
Mitochondria are important organelles because they generate most of the chemical energy needed to power a cell. The energy produced by mitochondria comes in the form of ATP or adenosine triphosphate, the energy currency that helps drive essential reactions and processes in living cells including cell signaling, muscle contraction, and active transport to name a few.The function of the mitochondrion is closely linked to the availability of oxygen. Mitochondria consume oxygen to convert the chemical energy from food into ATP by oxidative phosphorylation. In the matrix of the mitochondria, NADH is produced by the tricarboxylic acid cycle (Krebs cycle or citric acid cycle). The tricarboxylic acid cycle is used to generate ATP by enzymes that sit in the mitochondrial inner membrane.
Mitochondria are essential for health and longevity. Predictably, dramatic consequences arise when their function is compromised.
In this blog, we look at how microplate readers can be used to measure the “health status” of mitochondria and help researchers in their quest to produce new and better drugs for disease treatment.
Specifically, we provide practical examples of how the parameters of mitochondrial health can be measured in the context of mitochondrial toxicity.
Broadly speaking mitochondrial toxicity encompasses damage to the mitochondrion arising from disease or damage induced by external agents.1 Accordingly, mitochondrial toxicity is not only linked to disease, but it is also a major contributory factor to drug-induced toxicity.
Disruption of cellular energy metabolism significantly affects many diseases. The range is wide – from what are referred to as specific mitochondrial diseases to cancer, diabetes, neurodegeneration, and morbidity linked to cardiovascular health. The specific diseases of the mitochondrion, which include conditions like mitochondrial myopathies, belong to a group of genetic disorders that affect the function of mitochondria and may give rise to many symptoms including muscle weakness, seizures, vision impairment, or hearing loss. They are also associated with delays in development.
Mitochondrial dysfunction and toxicity also play roles in the development and progression of Parkinson’s and Alzheimer’s disease. Changes to the function of mitochondria can impact cell growth and proliferation in ways that lead to different types of cancer. In some cases, impaired insulin secretion and malfunction of the pancreas also arise from toxicity in the mitochondrion. The proper function of the heart and circulatory system requires a high energy demand which also means that mitochondrial dysfunction can have a significant effect on cardiovascular health.
Collectively, the impact of mitochondrial dysfunction on disease is wide and far reaching.
Today mitochondrial toxicity is a known side effect for many drugs. However, this was not always the case. Because mitochondrial toxicity earlier went undetected during drug development and testing, some drugs were by necessity withdrawn from the market and even received black box warnings. We now know that members of several drug classes cause mitochondrial drug toxicity and negatively affect mitochondrial function.
One example of the undesired impact of drugs on mitochondrial health is known from the history of drug discovery for acquired immunodeficiency syndrome (HIV/AIDS).2 Nucleoside reverse transcriptase inhibitors (NRTIs) are frontline drugs used as part of antiretroviral therapy (ART). This type of drug effectively interferes with the replication of viral DNA by inhibiting the enzyme reverse transcriptase.
Over the years, ART has significantly improved the treatment of HIV/AIDS transforming it from an acute to a chronically manageable disease. However, the first NRTIs developed for the treatment of HIV/AIDS were also associated with mitochondrial toxicity. This toxicity arose since this type of drug also inhibits the mitochondrial DNA polymerase, an enzyme necessary for the replication of mitochondrial DNA.
Today, mitochondrial toxicity is less common in newer versions of NRTIs including emtricitabine, lamivudine, and tenofovir. Once the effect of mitochondrial toxicity was known for this type of treatment for HIV infection, researchers found ways to minimize mitochondrial toxicity through improvements to drug design and increasing the selectivity for the viral enzyme. Reduced dosages and combination therapy also decreased the likelihood of mitochondrial toxicity. HIV infection is just one example where mitochondrial toxicity is a consideration for the treatment of diseases with a drug.
Overall, the main source of toxicity issues during drug discovery campaigns and clinical trials pertain to mitochondria.3-5 Screening for mitochondrial toxicity is therefore essential in the early stages of all drug development and is an important step in the assessment of drug safety for preclinical drug candidates identified through high-throughput screening efforts.
Researchers have different options when they want to assess mitochondrial toxicity. Oxygen consumption is one of the most informative and direct measures of mitochondrial function but is not the only option. Mitochondrial damage can also be assessed by looking at parameters such as mitochondrial membrane potential, adenosine triphosphate levels, extracellular acidification rates, reactive oxygen species, glutathione, and other viability assays. Such studies can be done with isolated mitochondria, permeabilized cells, living cells, tissue and animal samples.
A wide range of cell-based and cell-free assays, which are suitable for microplates, are amenable to these types of studies. Over the years, reliable, sensitive assays have been developed to measure mitochondrial toxicity that depend for the most part on detection by fluorescence, time-resolved fluorescence, phosphorescence, and luminescence. Microplate readers are a useful way to perform these assays at scale and offer the ability to perform multiplex analyses if needed. Here we look at how some of these assays are used starting with oxygen consumption.
The MitoXpress-Xtra and MitoXpress-Intra assays from Agilent permit real-time measurement of extracellular oxygen consumption for whole cells or isolated mitochondria. They are reliable methods to determine respiration rates for metabolic characterization and to evaluate mitochondrial toxicities in a high-throughput format.
In the application note “Real-time monitoring of intracellular oxygen using MitoXpress Intra” the MitoXpress-Intra assay was used to monitor intracellular oxygen in HepG2 cells on conventional microplates (fig. 3). The fluorescent probe provides real-time monitoring of intracellular oxygen within a cell monolayer. The assay provides a way to easily assess transient changes in metabolic activity as well as low O2 conditions (hypoxia) which are not possible using extracellular probes.
Intracellular oxygen in cell samples was measured using a BMG LABTECH microplate reader equipped with an Atmospheric Control Unit (ACU). The ACU module can regulate O2 (and CO2) and reproduce the physiological as well as hypoxic conditions needed for live cell-based assays. In this study, phosphorescent lifetimes were determined from ratiometric time-resolved phosphorescence intensities (in TR-FRET mode).
In the application note “Real-time measurement of intracellular O2 in mammalian cells” MitoExpress-Intra was used to look at how cytosolic O2 tensions significantly impact signal transduction and cell metabolism. The experiments were performed on a CLARIOstar® microplate reader equipped with an ACU. Measurements were made from the bottom of the microplate with dual-reads in TR-FRET mode and samples were delivered using onboard injectors. The MitoExpress-Intra probe was taken up by the cells during an overnight loading period and responded in real-time to any changes in intracellular oxygen concentration. The assays were suitable for both two-dimensional cell cultures and a range of three-dimensional systems. In this assay, oxygen quenches the phosphorescent emission from the probe in proportion to the concentration of oxygen.
More information is available in the MitoXpress-Xtra blog from BMG LABTECH. O2 consumption can also be measured as part of a multiplex analysis. The application note “The CLARIOstar® with ACU exposes cells to ischemia-reperfusion conditions and monitors their oxygenation” describes the parallel monitoring (multiplexing) of oxygen consumption, Reactive Oxygen Species (ROS), and Mitochondrial Membrane Potential (MMP). The intracellular probe was used to track oxygenation in the cell during the ischemia-reperfusion cycle in iPS-derived cardiocytes (Cor.4U cells) and in HepG2 cells (liver model). MitoExpress-Intra phosphorescence was measured in TR-FRET mode using the bottom optic.
MMP, an essential component of the process of energy storage during oxidative phosphorylation, can be used to assess mitochondrial function and health.
The application note “Measuring mitochondrial membrane potential using the FLUOstar® Omega microplate reader” describes the measurement of MMP in fibroblast cells. The fluorescence emitted by the tetramethylrhodamine methylester probe was directly proportional to the membrane potential measured in 96-well and 384-well formats.
In this study, the Omega series microplate readers could readily be used to measure MMP. In addition, prolonged low-dose treatment of healthy fibroblasts with a mitochondrial toxin caused a significant reduction in MMP. The ability to accurately measure MMP gives useful information about the general health and function of mitochondria. In particular, it provides a useful indicator of the overall function of the respiratory chain and the potential of the mitochondrion to generate ATP and provide energy for other cell components.
MMP was also measured in the application note “The CLARIOstar® with ACU exposes cells to ischemia-reperfusion conditions and monitors their oxygenation” as part of the multiplex analysis of oxygen consumption and ROS measurements.
ROS are a group of highly reactive chemicals derived from oxygen. Examples include superoxide, hydrogen peroxide, the hydroxyl radical and singlet oxygen. Due to their high reactivity, ROS can have a damaging effect on other molecules in the cell. The measurement of ROS is therefore a useful indicator of mitochondrial health.
A useful tool to monitor ROS and cellular redox changes is reduction-oxidation sensitive green fluorescent protein (roGFP). roGFP are genetically encoded biosensors that can be engineered to monitor different redox enzymes. How they work and how they are used in research are described in more detail in the BMG LABTECH blog roGFP: a biosensor for cellular redox states.
In brief, roGFPs are GFP molecules where two surface-exposed cysteines have been introduced into the β-barrel region at positions 147 and 204. roGFP has two different redox-dependent excitation peaks at 405 nm and 488 nm, and a single emission peak at 510 nm. The oxidation of cysteine results in an increase of the excitation peak at 405 nm. The reduction of cysteine increases excitation at 488 nm. The ratio between the emission at 510 nm and the excitation either at 488 nm or 405 nm is therefore an indicator of the relative amount of oxidized/reduced roGFP.
In the application note Real-time monitoring of genetically encoded redox probes in mammalian cell monolayers, roGFP was fused to the thiol peroxidase Orp1 to generate a probe for the ROS hydrogen peroxide. In this system, it was possible to monitor the response of the roGFP- Orp1 probe in a monolayer of confluent lung adenocarcinoma cells after addition of H2O2. Moreover, the probe could be used to assess the impact of potential inhibitors on cellular redox homeostasis. In the study, one compound was identified that significantly impaired cellular recovery from a H2O2 challenge in a concentration-dependent manner. This compound could be considered a candidate drug to sensitize cancer cells to chemotherapy or radiotherapy.
The application note Mitochondrial oxidant generation follows oxygen deprivation and re-oxygenation describes the use of a roGFP2 probe to investigate hypoxic and non-hypoxic conditions. The study uses the CLARIOstar® microplate reader with an atmospheric control unit. This roGFP2 sensor was used to probe H2O2 production in yeast at different levels of oxygenation.
In the application note “Chemiluminescence measurement of the generation of reactive oxygen species” low concentrations of ROS were detectable in Candida yeasts and blastomyces by means of lucigenin-dependent chemiluminesence. Measurements were made in luminescence mode. In C. albicans, mitochondria can generate extracellular released ROS. In addition, inhibition was observed with terbinafine, a known scavenger of free radicals.ROS were also measured in the application note “The CLARIOstar® with ACU exposes cells to ischemia-reperfusion conditions and monitors their oxygenation” as part of the multiplex analysis of oxygen consumption and MMP measurements.
The depletion of glutathione can be linked to drug-induced mitochondrial toxicity and provides an indicator of mitochondrial health. Glutathione protects the mitochondrion from the toxic effect of ROS. Lower concentrations of glutathione mean less protection from the negative effect of oxygen radicals.
roGFP genetically encoded biosensors are one way to look at the different levels of reduced and oxidized glutathione in the cell or mitochondria. In the following video, Professor Bruce Morgan from the University of Saarbrücken describes the use of the glutathione-roGFP fusion complex Grx1-roGFP to detect the glutathione-specific redox potential. In this study, roGFP2 was combined with glutaredoxin or glutathione to monitor redox enzyme activity. The results revealed that the mitochondrial and peroxisomal pools of glutathione are highly reduced in mitochondria in yeast which is like the situation found in the cytosol. This reduced pool of glutathione is actively involved in removing damaging ROS, particularly H2O2, from the mitochondria.
In addition to reduction-oxidation sensitive green fluorescent proteins, other assays are available to assess the levels of glutathione that allow for detection of this antioxidant tripeptide on microplates. For example, the sulfhydryl reagent 5,5’-dithio-bis(2-nitrobenzoic acid) or DTNB reacts with glutathione to form the yellow derivative 5’-thio-2-nitrobenzoic acid which can be measured by monitoring the absorbance at 412 nm.
Sensitive fluorometric assays are also available for glutathione that are amenable for analysis on microplates. One example uses the dye monochlorobimane which reacts with glutathione in the presence of the enzyme glutathione S-transferase. Monochlorobimane has negligible fluorescence when not bound to glutathione but emits a fluorescent blue signal at 461 nm when bound to glutathione if excited at 380 nm.
The extracellular measurement of the rate of acid production is another option to monitor respiration and provide an indicator of mitochondrial health. The application note “Measuring changes in cellular metabolism by monitoring extracellular acidification and oxygen consumption in real-time” is an example of how extracellular acidification can be determined on the CLARIOstar equipped with an ACU. Oxygen consumption and extracellular acidification were measured simultaneously in real-time under physiologically relevant concentrations. Extracellular acidification was determined using the pH-Xtra kit which permits direct, real-time kinetic analysis of extracellular acidification rates. The measurements were physiologically relevant, suitable for multiplexing, and easily used for non-adherent cells.
ATP is a useful indicator of the viability of mitochondria since it represents one of the end products of the activity of these energy factories. Monitoring ATP levels can also be used to look at the effect of a wide range of drugs and biological compounds on mitochondrial health as well as a barometer of mitochondrial toxicity during disease.
In the application note “Promega ENLITEN® kit performed on a BMG LABTECH microplate reader” ATP levels were measured beyond the limit of detection specified by the manufacturer of the kit. The performance of the ENLITEN® kit was linear over a range of 100 fmol/well to 0.195 fmol/well. Detection is by luminescence and provides high sensitivity for measurements of ATP concentrations.
The range of assays available to measure mitochondrial toxicity extends to biologically important inorganic ions. For example, measuring calcium retention is a good indicator of mitochondrial function and viability.
The application note “Calcium retention capacity assay evaluates inhibition of mitochondrial permeability transition pore” describes the use of the dye Calcium Green™ 5N to determine the calcium retention capacity (CRC) of mitochondria. Calcium Green™ 5N exhibits an increase in fluorescence emission intensity upon binding to calcium. The CRC assay is a high-throughput assay for the screening of mitochondrial permeability transition pore (MPTP) inhibitors. It can also be adopted for use with live permeabilized cells.
As is evident from this blog, researchers can choose from many available assay options to study mitochondrial toxicity. BMG LABTECH provides high-performance microplate readers that support the diverse applications that scientists wish to perform. Mitochondrial toxicity assays can be analyzed using the PHERAstar® FSX, VANTAstar®, CLARIOstar® Plus, or Omega series fluorescence plate readers in Time-Resolved Fluorescence, Fluorescence and Luminescence modes.
Collectively, these multi-mode readers combine high performance with miniaturized assays, short measurement times, and offer considerable savings on materials and other resources.
You can read more widely about metabolism in eukaryotic cells in the following blog from BMG LABTECH: cell metabolism.
Powerful and most sensitive HTS plate reader
Most flexible Plate Reader for Assay Development
Upgradeable single and multi-mode microplate reader series
Flexible microplate reader with simplified workflows
Gene reporter assays are sensitive and specific tools to study the regulation of gene expression. Learn about the different options available, their uses, and the benefits of running these types of assays on microplate readers.
Life in the depths of the ocean operates under extreme conditions. Find out how proteins from deep-sea luminescent organisms are useful for measurements on microplate readers.
Next generation sequencing (NGS) technologies for DNA or RNA have made tremendous progress in recent years. Find out how microplate readers can advance the quality control of nucleic acids to facilitate NGS.
Measuring light scattering is an accurate and expedient way to determine drug solubility. Find out how the NEPHELOstar® Plus can be used for early drug solubility screening at high throughput.
Find out how microplate readers can be used to measure histone deacetylase (HDAC) activity and assist drug discovery.