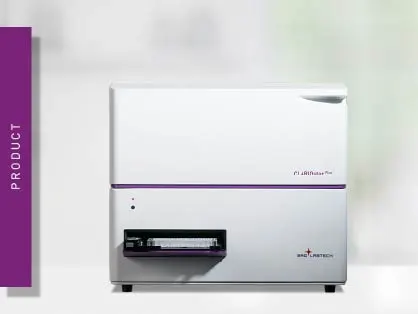
CLARIOstar Plus
Most flexible Plate Reader for Assay Development
Ischemia is the oxygen and nutrient deficiency that is triggered by vascular congestion. A sudden recovery is called reperfusion. How can this process be studied in vitro? You can find out in this blog post.
Ischemia-reperfusion (I/R) research studies the mechanisms underlying tissue and cellular damage caused by a period of ischemia, followed by the re-establishment of blood supply (reperfusion) in an infarcted organ or tissue.
Ischemia (also ischaemia) is defined as oxygen and nutrient deficiency and is typically caused by a restriction in blood supply triggered by vascular occlusion (fig. 1). The recovery of blood, oxygen, and nutrient supply are called reperfusion. I/R describes a cycle of restriction of blood (and oxygen) supply to tissues and subsequent return of the same.
I/R research focuses on several diseases but most notably on heart attacks and the myocardial tissue. Myocardial ischemia (cardiac ischemia) is the deprivation of oxygen and nutrients in the heart tissue. Myocardial ischemia takes place during myocardial infarction, where vascular congestion, typically caused by an occlusive thrombus in a coronary artery, impedes blood supply to the myocardial tissue (fig. 2). Subsequent vascular decongestion (reperfusion) restores blood supply to the ischemic myocardial tissue, however paradoxically causing additional tissue damage.
I/R research focuses on the study and identification of the underlying causative molecular mechanisms to avoid the damaging effects of both ischemic and reperfusion injury, with a special focus on the myocardial tissue and on myocardial infarction. In the last decades, I/R research has helped to expand our understanding of the disease and the injuries that it causes. However, even today the tools and models to study this phenomenon in a laboratory environment are limited.
Although mainly associated with myocardial infarction, ischemia-reperfusion injury is also associated to stroke (brain ischemia), cutaneous, mesenteric, or kidney ischemia, cancer, and a variety of diseases in different organs (fig. 3). It is a ubiquitous concern in organ and limb transplantation as well as in cardiovascular and reconstructive surgery.
The pathophysiological processes of I/R injuries are both systemic and local and can be divided into two phases. While the depletion of the cellular energy metabolism is the main effect during the first ischemic phase, inflammation, oxidative stress, and apoptosis are predominant during reperfusion (second phase).1
Depending on the affected tissue or organ, ischemia-reperfusion injury is associated with heart failure, cerebral dysfunction, systemic inflammatory response syndrome, and multiple organ dysfunction.
2.1 Ischemia: cellular effects
During the ischemic stage, the deprivation of oxygen and nutrients caused by a deficiency of blood supply severely affects cellular energy metabolism. Sensitivity to hypoxia varies among different cell types, depending on how metabolically active they are. However, cellular damage is always related to the persistence (time) of ischemia.
Amongst the different cellular compartments, the mitochondria and the endoplasmic reticulum are affected the most by ischemia, mainly because of their capability to sense oxidative stress, maintain ion homeostasis, and trigger apoptosis.1 In particular, mitochondria play a central role in energy production and are considered a druggable target to counteract I/R injury.
Oxygen shortage inhibits oxidative phosphorylation-dependent ATP production and negatively affects mitochondrial coupling, inhibiting the electron transport in the mitochondrial respiratory chain. Accordingly, the cell switches to anaerobic metabolism. During anaerobic metabolism, lactate, hydrogen ions, succinate, and alanine are increased while glucose, glycogen, and glutamate production decrease. This condition reduces the extracellular and intracellular pH, affects ion homeostasis, leads to membrane depolarization, and intracellular Na+ and Ca2+ accumulation (fig.4).1
Mitochondrial disfunction triggers apoptosis2, eventually resulting in necrosis and irreparable tissue damage. 3, 4 Tissue damage induces the production and release of cytokines and consequent systemic inflammation.
2.2 Reperfusion injury: cellular effects
Tissue ischemia is frequently associated and related to reperfusion injury. In fact, experimental evidence indicates that tissue and cell damage are mostly associated with the restoration of blood flow upon ischemia than with the ischemic phase itself.
The restoration of blood supply increases the levels of circulating free radicals (ROS) and mobilises platelets, T-lymphocytes (CD4), and neutrophils in the vascular space of the hypoxic tissue. This recruitment aggravates tissue damage through the activation of an inflammatory response and the production of inflammatory cytokines such as TNF-α, TNF-β, interferon-gamma, and macrophage-stimulating factors. 1
Pro-inflammatory cytokines directly or indirectly induce oxidative stress through the production of reactive species (ROS), such as hydrogen peroxide and superoxide anion, and trigger an increase of endothelial adhesion molecules. 1, 3, 4 Here, a pivotal role is played by mitochondria as an intracellular source of ROS. This combined with reduced nitric oxide levels leads to vasoconstriction, micro-circulatory insufficiency, and prolonged ischemia (fig.4). 5
At the cellular level, damage induced by prolonged ischemia-reperfusion injury leads to apoptosis, autophagy, and necrosis. Moderate reperfusion injury may cause autophagy and activate recovery survival systems.
All pharmacological approaches developed through I/R research try to block or limit the damaging process by targeting key cellular events, such as the limitation of free-radical production by scavenging, the blockade of neutrophil recruitment, and activation, or the impairment of endothelial relaxation. 7 However, the mechanisms of injury are multifactorial and involve several signalling pathways.
Possible pharmacological targets include the hypoxia-inducible factor (HIF) pathway, the Reperfusion Injury Salvage Kinase (RISK)8, the AMPK pathway9, the antioxidant transcription factor Nrf210 and autophagy, necrosis, and apoptosis pathways11. In addition, the mitochondrial permeability transition pore is considered an important target.
Despite the progress achieved in understanding its pathophysiology, the establishment of new treatments for I/R injuries still represents a major challenge. Indeed, I/R research is still failing at translating research results from bench to bedside. Because of its complexity, only a few treatments have reached clinical phase III trials.
In I/R research, experimental models have provided considerable advances in understanding its mechanisms, as well as in testing new strategies to reduce tissue damage. Because of its relevance, most studies target the heart and myocardial tissue.
3.1 In/ex vivo models
Currently, I/R research primarily relies on small animal models to clarify the molecular mechanisms underlying injury. Animal models are useful as they enable the examination of the interactions between various cell types during injury. However, they also introduce several variables that can complicate the analysis of the effects exerted by specific compounds. In animal models, myocardial injury is typically simulated by using a suture to occlude a coronary artery and then by releasing the suture to allow for myocardial reperfusion.
Researchers have also explored the use of isolated perfused hearts (Langendorff heart or isolated perfused heart assay). Although this model allows for a more controlled environment, it does not fully recapitulate human physiology.
Although physiologically relevant, in vivo and ex vivo approaches are limited in throughput, are expensive, and require skilled surgeons.
While animal models remain critical for drug development, in vitro models are an important alternative as they can highlight the direct effect of reperfusion itself and of a drug on specific cells (e.g., myocardial cells), isolating and individually controlling any external factor.
3.2 In vitro models
There are different ways to mimic ischemic and reperfusion injury in vitro. Cells are typically seeded in 6 – 24 well microplates and placed in a hypoxic chamber, a gas-tight chamber that is flushed with nitrogen to achieve a hypoxic environment. Microplates are then incubated for 1 - 20 hours, depending on the cell type.
In this model, cells are typically cultured in media lacking metabolic substrates. Moreover, additional potassium is supplemented to achieve hyperkalemia, and lactate is added to mimic its accumulation due to anaerobic glycolysis and to acidify the pH. 12
The disadvantage of this model is that researchers can neither follow the cellular behaviour in real-time nor apply additional handling (e.g., treatments) as the samples are not accessible.
To mimic reperfusion, cells are brought back to physiological oxygen tension, the “hypoxic medium” is discarded and replaced by a full growth cell culture medium for nutrient delivery. Cells are left in this environment on average for four hours, however, this depends on the cell type.
Placing cells with a minimal amount of medium under a layer of mineral oil is an alternative method to achieve hypoxia. The oil seals the sample and blocks the diffusion and exchange of oxygen, this way achieving a gradual reduction of nutrients and accumulation of wastes.
Despite their advantages, these methods still provide limited throughput, and the amount of obtainable information is quite poor.
Different microplate readers from different manufacturers offer the possibility to control the environment inside the reader. Typically, CO2 and O2 can be controlled in a range from 1 - 20%. In some cases, readers can go down to 0.1% O2. Atmospheric control obviously facilitates I/R research as plate readers can increase throughput (plate compatibility is up to 1536-well plates in some cases) and enable kinetic data acquisition in real-time.
Nevertheless, these plate readers can only actively decrease O2 through the purging of nitrogen. Recovery to normoxia is achieved through passive diffusion of the excess nitrogen out of the reader. Accordingly, conventional plate readers can only cover the ischemic phase and are not suited to mimic reperfusion injury.
The only exception is the CLARIOstar® Plus microplate reader with Atmospheric Control Unit (ACU).
Mimicking ischemia-reperfusion
In fact, the ACU of the CLARIOstar Plus reader was developed to support research on the biological effects of oxygen dysregulation. In addition to sustaining hypoxic and ischemic conditions, rapidly decreasing oxygen up to 0.1%, the ACU can also rapidly re-oxygenate the measurement chamber of the CLARIOstar Plus to simulate reperfusion.
The course of oxygen and carbon dioxide concentrations is independently programmable by the user. The profile of oxygen deprivation and re-oxygenation is executed automatically by the ACU while the biological impact is recorded by the microplate reader (fig. 5).
The unique ability of the CLARIOstar Plus with ACU to mimic myocardial I/R conditions can be used to screen in high throughput for compounds that can protect against myocardial damage upon a heart attack. Dr. Ben Allsop, the Research Associate at the University College London, UK, discusses this topic in the scientific talk: “Simulating ischemia-reperfusion injury in vitro as a potential method for drug screening.”
In his talk, he also compares the different myocardial injury models, demonstrating that the CLARIOstar Plus with ACU reaches 0.1 % O2 in around 45 minutes, much faster than any other system (e.g., a hypoxic chamber requires 120 minutes to get to 1% O2). Further advantages of this system include a reduced cost and size of animal studies as a consequence of efficient in vitro compound selection, lower skill requirements compared to myocardial surgery in animal work, and the possibility to incorporate iPSC cardiomyocytes.
Another example is shown in the following application note: Lipid peroxidation assay in an ischemia-reperfusion cell model with NCLX inhibitors using the CLARIOstar® Plus. Ischemia reperfusion events lead to an increase in lipid peroxidation events in cells. In this example the CLARIOstar Plus with ACU was used to simulate ischemia (1% O2) and reperfusion (18% O2) to study potential inhibitors of lipid peroxidation in neuroblastoma cells using a fluorescent probe.
The oxygen ramping feature enables in vitro studies of I/R in high throughput and in real-time. The possibility to conveniently analyse multiple parameters in parallel (multiplexing) ensures the maximum output of each experiment, as shown in the app note “Mitochondrial oxidant generation follows oxygen deprivation and re-oxygenation.”
Moreover, the CLARIOstar Plus with ACU can replicate physiologically relevant in vivo oxygen conditions to increase data significance. Cell-based assays are typically run at non-physiologically relevant O2 levels such as 21%. This topic is discussed by Prof. Giovanni Mann, Prof. Vascular Physiology at King's College London, UK, in the scientific talk: “Defining physiological normoxia in cultured cells for translation to animal models and man.”
Prof. Giovanni Mann shows the impact that working under physiological conditions can have on improving the interpretation of data, and explores how and why O2 as a physiological parameter can influence data.
Most flexible Plate Reader for Assay Development
Atherosclerotic vascular disease is one of the leading causes of death globally. Here we explore some of the research options available to scientists to tackle this significant threat to public health.